The city of Beirut sits on a peninsula along Lebanon’s Mediterranean coast. The Port of Beirut, opened in 1887, is located directly north of downtown Beirut and mostly separated from the commercial and residential quarters by the Charles Helou highway. The port contains berths for freight vessels, grain silos, and storage hangars. Over the years, thousands of tons of ammonium nitrate ended up being stored in Hangar 12, located directly east of the grain silos. For yet unknown reasons, the same hangar stored fuel, acid, fuse spools, and 15 tons of fireworks. On Aug. 4, 2020, at about 6:07 p.m., a sequence of events started a chain reaction by which a fire in the hangar caused the fireworks and subsequently the ammonium nitrate to detonate, resulting in nearly 200 deaths, more than 6,000 injuries, about 300,000 people losing their homes, and $10 billion to $15 billion in damage throughout the city. Several damaged buildings were assessed in the weeks following the explosion, and in the following article, we compare their actual damage with that predicted by industry standards. Our aim is to further engineers’ understanding of the accuracy of current explosive safety models and analyses. Underlying this effort is one question: What can we learn from the Beirut explosion to better prepare other urban environments for accidents such as this?
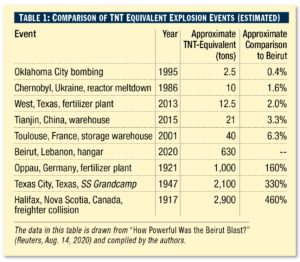
Explosion Event and Magnitude
On Aug. 4, 2020, just after 6 p.m., an intense fire broke out at Hangar 12 in the Port of Beirut. Unbeknownst to the nearly 100,000 people who lived within 1.6 km of the warehouse, Hangar 12 was storing everything needed to make a bomb. The fire started a chain reaction, beginning with the activation of fireworks and finishing with the detonation of ammonium nitrate. According to researchers, approximately 2,750 tons of ammonium nitrate were stored in Hangar 12. Based on eyewitness videos and estimated shock wave velocity, researchers and experts have estimated that approximately 1,500 tons of ammonium nitrate contributed to the explosion. In practice, explosions are defined based on their equivalence to a TNT charge to better match historical experimental data and use industry standard relationships between the TNT charge weight and the pressure/impulse measures that are used for analysis purposes.
The explosion resulted in a form of blast wave that, although not unexpected, was considerably different in duration from smaller explosive events. To put the Beirut explosion into perspective, Table 1 compares the TNT equivalent with other historical explosion events. For the purposes of this article, the authors use a TNT equivalent of 630 tons, although current estimates vary from 300 to 960 tons.
Industry Standard Methodology
It is important to understand how industry standard calculation methods compare to the damage witnessed throughout Beirut. The U.S. Department of Defense Explosives Safety Board and the United Kingdom Ministry of Defence publish standards on proper siting for explosive safety. The siting requirements include minimum distances between the stored explosives (Hangar 12 for this event) and inhabited buildings, public traffic routes, electrical systems, and other explosive storage locations. The resulting distances are based on the quantity of stored explosives and construction of the storage buildings (i.e., earth-covered bunker versus light-gauge warehouse). In general, the goal of explosive safety siting is twofold: life safety for the public and mitigating the propagation of explosives between storage locations. The critical parameter most applicable to Beirut is the inhabited building distance. The IBD is the minimum permissible distance allowed between a quantity of explosives and any building inhabited by the public or where people are accustomed to assembling. This minimum distance provides a high degree of protection against structural damage based on blast or shock wave effects to frame or masonry buildings. It does not provide protection against glass breakage. Personal injury from flying glass is possible even when in compliance with government regulations due to its high failure potential under low blast pressure. The IBD for the assumed 630 tons of TNT equivalent explosion is a distance of 1,700 m (according to U.S. standards) and 1,900 m (according to U.K. standards), which results in a peak pressure of 6 kPa and 5 kPa, respectively. The expected pressure at the IBD is sufficient to shatter typical residential and commercial glazing, which is considered allowable damage.
Structural response to explosive events has been studied in extensive detail since World War II. The Jarrett damage criteria for buildings, published as Derivation of the British Explosives Safety Distances in the Annals of the New York Academy of Sciences in 1968, are common references when considering explosion effects. These damage criteria can be further developed into pressure-impulse or iso-damage curves, as shown in Figure 1. PI curves allow an estimate of building damage based on the peak side-on overpressure versus impulse from an explosive event. Examination of these iso-damage curves shows that as the peak pressure load and impulse increase, the corresponding level of damage also increases. However, these iso-damage curves also indicate that there are different regions of loading response: impulsive, quasi-static, and dynamic, also known as pressure-time. In the impulsive region, the structural response is controlled by the overall energy of the blast wave above a threshold peak-pressure level. In the quasi-static region, the structural response is governed by the peak pressure almost regardless of the impulse. In between, the dynamic region response is dependent on both the peak pressure and the impulse.
A key characteristic of the pressure wave from an explosion is the relationship between the peak pressure and impulse with range. As the pressure wave from an explosion expands, the resultant pressure wave peak decays rapidly and the duration of the blast wave increases. Explosions in the impulsive region tend to occur from smaller explosions, close to the target, whereas explosions in the quasi-static region tend to be the result of extremely large explosive events. Figure 2 shows the Jarrett PI curve with a range-charge weight overlay of three charge weights (630 tons TNT, 500 kg TNT, 1,000 kg TNT) clearly showing the different response regions.
Damage Assessment
It is also important to quantify the damage throughout Beirut to compare it with the explosive safety and blast-loading methodology. It is difficult to personally investigate the entirety of damage in the city, so the damage was quantified and compared using various methods as shown in Figure 3. Initially, the Earth Observatory of Singapore, in collaboration with the Advanced Rapid Imaging and Analysis team at NASA’s Jet Propulsion Laboratory and the California Institute of Technology, provided a high-level survey of damage using satellite imagery before and after the explosion. This damage map overlay uses differences between the satellite imagery to identify locations where damage is expected to be heavy (red) to moderate (yellow), with the absence of color signifying minimal damage. IBD curves corresponding to the range of TNT equivalent estimates are also plotted in Figure 3 for direct comparison to the industry-accepted explosive safety standards.
Subsequently, researchers and engineers from the American University of Beirut personally inspected and assessed individual structures throughout the city to quantify damage. The damage levels were broken down into similar categories as the satellite imagery, from destructive damage (red) to nondestructive damage (green). However, unlike the satellite imagery, the AUB team was able to differentiate between structural and nonstructural damage on a case-by-case basis. The individual buildings assessed by the AUB team are shown in Figure 3 using appropriately colored circles.
Beirut features a variety of ancient religious buildings as well as historic, heritage, and newly developed buildings and high-rises. Most of these buildings were affected by the blast waves, which caused significant or partial damage. The level of damage is mainly dependent on the type, age, and location of these buildings. The damage assessment for these buildings is being performed to inform the public about the extent of damage that occurred and to assist in the engineering decision of either repairing or demolishing the affected buildings. Also, these assessments help provide the cost estimations for buildings that need to be repaired.
For residents, Beirut now looks like a war zone, with broken glass, destroyed facade systems, and collapsed buildings at every turn. But the city is also now a treasure trove of data for engineers who study the effects of explosions on structures and infrastructure. To help the city’s recovery efforts, researchers and engineers at AUB set up a hotline for residents or building owners to schedule structural damage assessments. The initial observations from these assessments show that damage can be classified, and the buildings tagged, using three main categories: destructive (red), partially destructive (yellow), and nondestructive
(green).
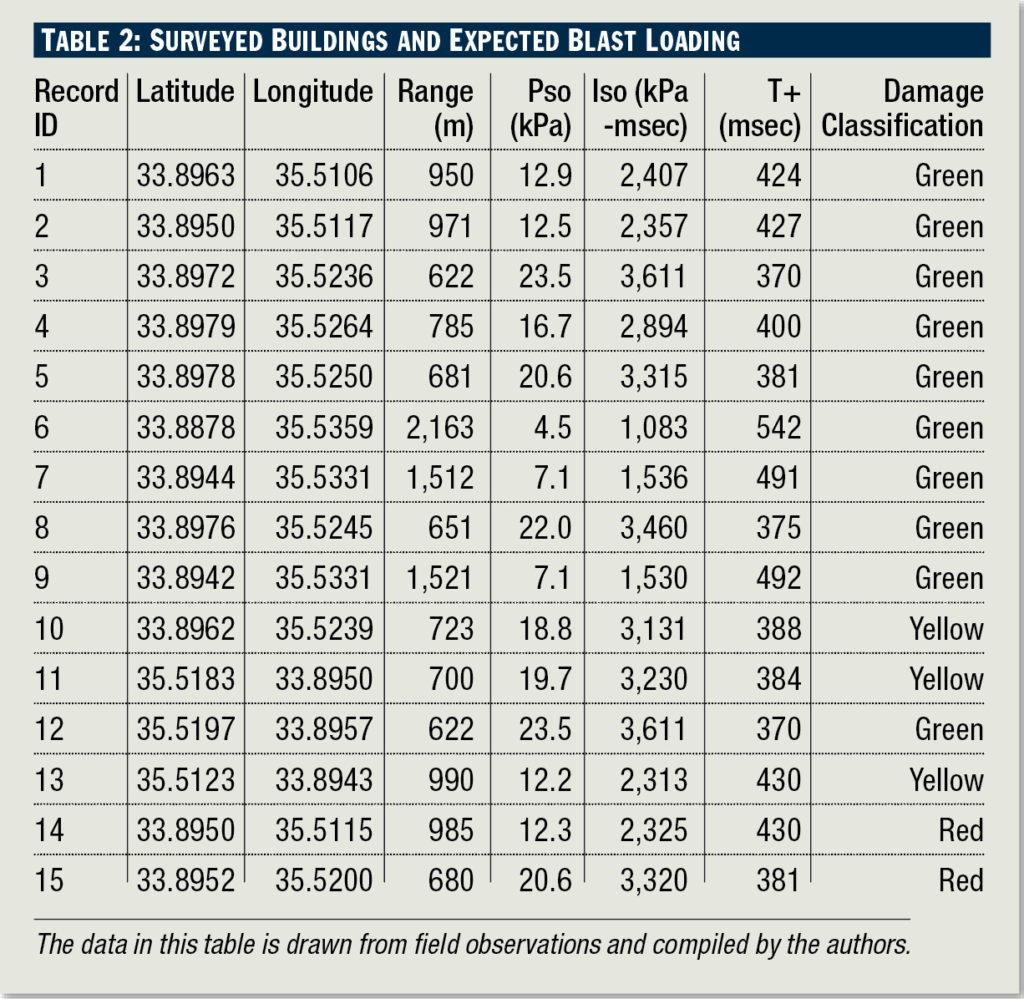
Table 2 details a subset of the buildings that the group surveyed, the range from the explosive event, and the peak side-on overpressure and impulses based on a 630-ton TNT equivalent blast that the authors calculated using the Kingery and Bulmash equations described in the U.S. Army Ballistic Research Laboratory’s Airblast Parameters from TNT Spherical Air Burst and Hemispherical Surface Burst, Technical Report 02555, published in April 1984.
The buildings exhibiting destructive damage collapsed, as did the unreinforced masonry bearing wall of the building. This building is located about 1 km away from the explosion, and it is classified as a historical masonry building that consists of unreinforced masonry blocks from natural stones with wooden roof trusses. This type of building is designed to resist gravity and wind loads only; it cannot resist lateral loads (that is, earthquake or blast loading). This is due to the absence of seismic structural systems and horizontal diaphragms that can help in resisting the shock waves due to explosion. A reinforced-concrete building located near the collapsed historical building withstood the blast load without any severe structural damage recorded. It is assumed that the gravity reinforced-concrete building frame provided adequate stiffness capable of resisting the blast shock waves.
The buildings identified as having partially destructive damage are not totally collapsed but were still severely affected by the explosion. These buildings need careful damage analysis and assessment to assist in the engineering decision to rehabilitate or demolish each structure. The image below shows a building identified as having partially destructive severe damage. The building is classified as a historical building that consists of natural stone masonry bearing walls with wooden roof trusses. This building is located about 1.2 km away from the explosion. The top timber roof truss failed and is sitting on the floor below. This is because the timber roof trusses, as constructed, cannot act as diaphragms to resist any lateral load. The third floor of the exterior wall of this building also experienced severe damage.
The buildings with nondestructive damage resisted the blast load with nonstructural component damage only. Those buildings were typically reinforced-concrete buildings located less than 1 km from the explosion. The damage was limited to window glazing, facades, external tiling, false ceilings, and water pipes and other utilities. Theses building were designed to resist earthquakes using reinforced-concrete shear wall systems that provide the building with very high stiffness in both directions, which was able to resist the blast-induced lateral loads. While this damage may seem minor with respect to the structural damage experienced by other types of buildings, it can severely impact a community’s ability to recover and occupy buildings damaged in this manner.
Mitigation of Future Events
For engineers, the obvious question is: What’s next? What can the building industry do to prepare for events such as this? The Port of Beirut explosion unintentionally resulted in a substantial amount of experimental data to be used in comparing expected and actual performance of buildings under an extreme blast event. The assessment and comparison methods discussed throughout this article only scratch the surface. However, four important findings were developed from this study.
First, explosive safety standards adopted by the United States and the United Kingdom were compared to actual damage witnessed from satellite imagery and field observations. In general, the existing minimum distances specified in government standards for the location of inhabited buildings are quite conservative, as nondestructive damage was routinely found within this limit. The IBD is typically applied to military bases or rural settings where sufficient space is provided throughout the site. Explosive safety standards are rarely applied to urban environments like that of Beirut.
Second, it is clear that there was an overall lack of structural damage in Beirut. Several old buildings, designed and constructed prior to modern seismic guidelines, did collapse. However, most buildings experienced substantial nonstructural facade damage. The failure of the facades helped dissipate the blast energy and kept the load from transferring to the structural load-bearing elements. Had this not occurred, the energy from the explosion could have caused substantial structural damage. It is important to note that lack of structural damage does not guarantee an adequate level of life safety. Broken glass, failed facades, damaged false ceilings, and inoperable elevators can and will present substantial dangers to building occupants. In fact, the Explosives Safety Board states in its Defense Explosives Safety Regulation 6055.09 that “it is evident that, even at IBD, conventional structures may not provide complete protection from the blast. Generally, the weakest portions of any conventional structure are the windows.”
Third, the damage in Beirut fits within the explosive safety framework commonly used throughout the world to protect people and infrastructure from extreme explosive events. Viewing the explosion and aftermath within an explosive safety framework highlights the wide variation of building response found throughout the city. While facade and nonstructural damage were found within the IBD, no structural damage was found outside of it. This means that a vast majority of the buildings in Beirut south of the Charles Helou highway survived the explosion with little to no structural damage, regardless of their proximity to Hangar 12. Had they not, the casualties would have been substantially higher and recovery for the city would take much longer. It is important to note that the buildings north of the Charles Helou highway, located at or directly adjacent to the Port of Beirut, were inaccessible to the AUB team. Observation of published photographs shows that this area was mostly destroyed.
Finally, the field observations and structural assessments are extremely important in helping provide engineering solutions for the damaged buildings in Beirut, especially for the ancient religious, historical, and heritage buildings. The structural design (i.e., seismic resistance) and construction quality vary throughout the infrastructure in Beirut, which has a major influence on buildings’ responses during explosions. Upgrading critical infrastructure and culturally important buildings susceptible to dynamic loading (seismic or blast) would help mitigate risk in future events.
Kevin Mueller, Ph.D., P.E., M.ASCE, is a senior project engineer in the Chicago office of Thornton Tomasetti. Elie Hantouche, Ph.D., A.M.ASCE, is an associate professor of structural engineering at the American University of Beirut. Nicolas Misselbrook, CEng, C.Phys, is a principal in the Dalgety Bay, Scotland, United Kingdom, office of Thornton Tomasetti Defence Ltd.
The authors would like to thank the AUB post-disaster building structure safety team, including the structural engineering faculty and staff and the university’s ASCE student chapter, for participating in the data collection.
This article first appeared in the November 2020 issue of Civil Engineering as “Lessons Learned.”
Errata: The print version of this feature incorrectly noted in the text that Figure 2 included a charge weight of 100 kg TNT. That number, as identified in Figure 2, is 1,000 kg. The print version of table 1 incorrectly listed Chernobyl as being in Russia; this version corrects the location to Ukraine. We regret the errors.
really like how this paper breaks everything down !
Well Written.
very effective articles for any civil engineers and whom are working with port authority.