The U.S. Army Corps of Engineers is in the midst of a decades-long effort to safely decommission and dismantle a trio of Army nuclear reactors. The first part of the project involved the successful deconstruction of a nuclear power plant onboard a ship — which provided the Corps with multiple ‘lessons learned’ to improve the process for the remaining two land-based reactors.
In April 1957, the U.S. Army activated the first nuclear power reactor in the United States that provided electricity to the commercial power grid for an extended period. Based at Fort Belvoir in Fairfax County, Virginia, just outside Washington, D.C., the SM-1 reactor was a prototype of a small nuclear power plant primarily intended as a training facility for reactor operations personnel from the Army, Navy, and Air Force. But its roughly 10 MWt capacity was connected to the local power grid in Northern Virginia and preceded the nation’s first strictly commercial nuclear power plant, which was constructed in Shippingport, Pennsylvania, in 1958.
The SM-1 was part of the Army Nuclear Power Program, a joint effort between the U.S. Army Corps of Engineers and the Atomic Energy Commission to develop “small, rugged, and transportable nuclear plants that could provide both heat and electricity at remote military installations,” according to the Corps’ online history of the program. Following its success with the SM-1, the Army Nuclear Power Program designed, built, and operated another seven reactors in the United States and at sites around the world. These included the 20.2 MWt SM-1A at Fort Greely, Alaska, in 1962, and the 45 MWt MH-1A in 1967, which was constructed inside the Sturgis, a former World War II Liberty-class cargo ship that was converted into a floating nuclear power barge to help fulfill the program’s goal of producing mobile reactors.
In the 1970s, when they ceased to be necessary, three of these reactors — Fort Belvoir’s SM-1, Fort Greely’s SM-1A, and the Sturgis’ MH-1A — were deactivated and all their uranium nuclear fuel and control rods were removed as the first step toward decommissioning and dismantling the facilities, says Brenda M. Barber, P.E., a program manager with the Environmental and Munitions Design Center in the Corps’ Baltimore District. At that point, control of the three reactors switched to the Army Deactivated Nuclear Power Plant Program.
The process of decommissioning and dismantling a nuclear reactor is not simple — and it certainly is not quick. After the removal of the fuel and control rods, as well as any liquid waste at the sites, each of the three deactivated reactors was then essentially mothballed — put into what the Corps refers to as SAFSTOR, for safe storage, Barber says. That SAFSTOR period lasted roughly 40 years so that the radioactivity of the various remaining reactor components could decay, eventually enabling the decommissioning crews to “safely perform the work without (exposure to) any significant doses of radiation,” she explains.
The Army’s reactor program is self-regulated, Barber adds, but follows the guidelines of the Nuclear Regulatory Commission, which set a 60-year time frame for how long it can take between when a reactor is taken offline and the date by which it must be dismantled and its site made safe for reuse or other activities. The Sturgis reactor was the first to be completely dismantled, beginning in 2015 and ending last year when the ship itself was broken up for scrap. Fort Belvoir’s reactor is scheduled for dismantling next, the work expected to begin early in 2021 and take roughly five years to complete. Work on Fort Greely’s reactor could begin in 2022 but might take as long as eight years to complete due to the remoteness of the site — about 100 mi southeast of Fairbanks, Alaska — the potential for harsh weather conditions, and various other complications, Barber says. The difficulty of accessing Fort Greely was one reason the site was chosen for a reactor in the first place, Barber notes, because it was sometimes problematic to keep the base supplied with regular shipments of conventional fuels.
At all three sites, the remaining low-level radioactivity comes from the metals used in what remains of the reactor systems. The two major radioactive elements of concern are Nickel-63 and Cobalt-60. Cobalt-60 is a gamma-emitting isotope with a decay half-life of about five years while Nickel-63 is a low-emitting beta isotope with a decay half-life of 100 years, making it a long-term concern for disposal, Barber notes. More than 99 percent of the Cobalt-60’s radioactivity had decayed during the time in SAFSTOR, which makes the decommissioning safer for the teams from an occupational and radiological safety perspective, Barber explains.
The Corps had dismantled at least two small research reactors before the start of the current program, Barber says, and it has relied on the expertise of private contractors from the commercial nuclear reactor sector as well as other branches of the military and other government agencies with similar experience. Most importantly, perhaps, “we learned a lot of very hard but good lessons” during the Sturgis dismantling that will help improve the processes at Fort Belvoir and Fort Greely, she explains.
Before becoming a nuclear power plant barge, the Sturgis had been a 14,000-ton World War II cargo ship named the S.S. Charles H. Cugle. Measuring more than 441 ft long, the ship was converted to a floating nuclear barge by the removal of a 212 ft long section in the middle of the vessel, a section that included its engine and propulsion systems; as the Sturgis, it had to be towed wherever it was taken, hence its designation as a barge. A new, wider midsection was then installed and structurally joined with the watertight bulkheads of the original ship’s bow and stern sections. The nuclear reactor was located in the center of the vessel to protect it from “potentially catastrophic impacts, such as might occur with a severe grounding of the vessel or a broadside collision with another large vessel, and consequential sinking of the vessel,” according to a July 2019 historical mitigation report, Deactivated MH-1A Nuclear Power Reactor and Barge STURGIS. The report was prepared by Horizon Environmental Services Inc. and Gray & Pape Inc. to document the project for the Corps and the prime contractor, APTIM Federal Services LLC.
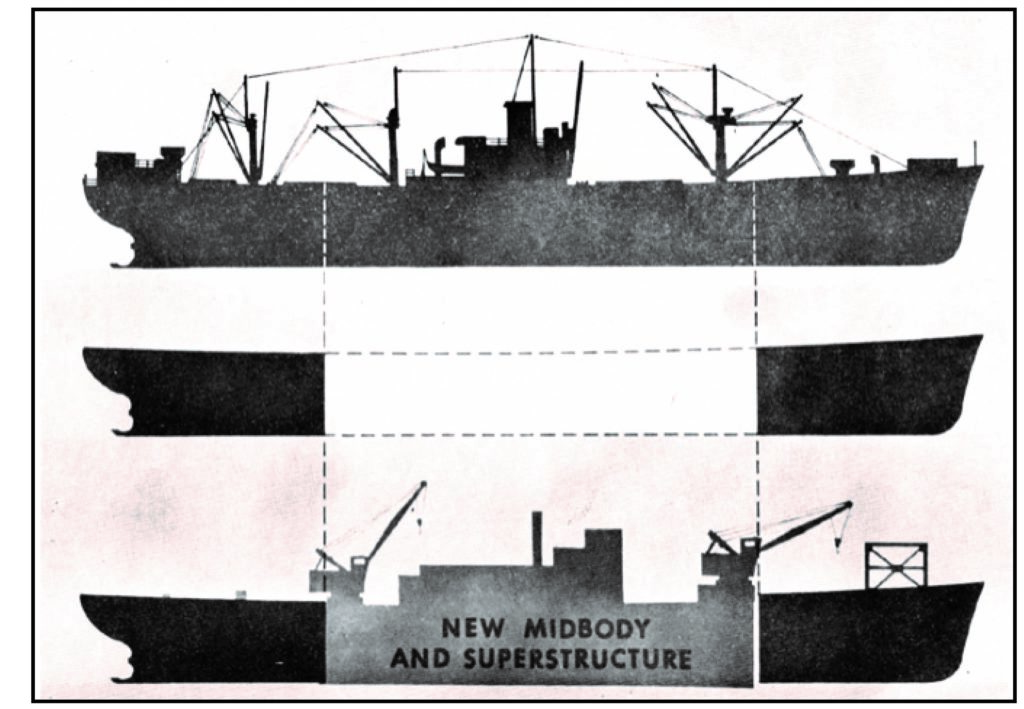
Additional protection for the reactor included a lead-lined sphere that housed the reactor components surrounded by steel-reinforced concrete walls. The concrete walls made the new midsection 8 ft wider than the original beam of the vessel, requiring the fore and aft sections to be tapered to accommodate the new width, according to the mitigation report. A new inner hull section was also created and filled with compartments for saltwater, freshwater, and other liquid ballast to provide 7 ft of separation between the reactor deck and the hull.
The Sturgis spent most of its useful life in the Panama Canal Zone, providing power for military and civilian purposes. After being towed to Fort Belvoir — on Gunston Cove, off the Potomac River — for the initial removal of the nuclear fuel, control rods, and radioactive wastes, the Sturgis was towed to the James River Reserve Fleet at Joint Base Langley-Eustis, near Newport News, Virginia, for long-term storage and monitoring. By April 2015, quarterly monitoring by the Corps indicated that the radiation levels within the Sturgis had decayed sufficiently to begin dismantling. At this point, APTIM (then known as Chicago Bridge and Iron, or CB&I Capital Services) conducted a laser scan to collect point-cloud data that was used to create 3D images and models of the inside of the reactor access compartment and the reactor containment vessel areas, which is where the components of the former reactor were located.
“The modeling allowed us to simulate a task without needing to have personnel go into the radiological areas until we had confidence in our approach and we had verified potential doses,” says Barber. “While we had reports and other information to help us paint a picture of the inside, we did not have access, and this imagery was invaluable in planning for the decommissioning and dismantling by giving us firsthand knowledge of the size, location, and dimensions of components we would be handling. This was accomplished without needing to breach the containment structure or have personnel spend extended time within the containment structure, which was home to the vast majority of the Sturgis’ radioactivity.”
The Sturgis was then towed on a roughly three-week journey to the Malin International shipyard at the Port of Galveston in Texas, where the dismantling work would take place. To prepare the site, Malin hired additional employees, including security guards, and APTIM installed security fencing and electrical services for the barge, among other work. “Out of an abundance of caution,” Barber adds, “we reinforced the dock in Galveston” to accommodate the heaviest materials that would be removed from the Sturgis. The barge’s onboard cranes were refurbished, new boarding ramps were acquired to access the barge from the dock, and a ventilation system was established including high-efficiency particulate air filtration to ensure that “all radiological work” would be performed “under negative pressure during decommissioning efforts,” according to a Corps website that was used to provide updated information to the Galveston community and other stakeholders throughout the Sturgis decommissioning process. Due to the extreme heat in the Galveston area, an air-conditioning system was also installed for the primary work areas on the Sturgis.
Along with the potential for radiation exposure, the work crews faced exposure to lead (including lead-based paint), asbestos, polychlorinated biphenyls, and other hazardous materials used in the wall, floor, and ceiling tiles and various other systems and components found throughout the Sturgis. It was these more traditional environmental concerns, not radiological safety issues, that required workers to wear protective clothing and respirators, Barber adds. The project team also trained with local emergency responders, including the police department, fire department, and other agencies, and maintained regular communications with these groups to ensure the safety of the work site and the community.
One of the first things the work crews did was design and engineer new openings in the deck of the Sturgis to provide access to the reactor and its components. These large penetrations had to be closable and weather tight to accommodate the potential for severe weather in the Galveston area, Barber notes. Moreover, the project team developed a hurricane plan that included securing the vessel itself and all openings; tightening the mooring lines, which were designed to withstand Category 5 storm winds; and removing any loose equipment, including the access ramps. The plan also involved close coordination with the Port of Galveston and the U.S. Coast Guard. Such preparations proved their worth in late August 2017 — while the deconstruction work was well underway — when Hurricane Harvey approached the Texas coast, shutting down the Sturgis project until early September. But everything worked out well. “The site remained secure and did not suffer damage during the storm,” Barber notes, with “no evidence of releases of radioactive material (or) lead or increased radiation exposure.”
The new openings in the deck provided access to the upper refueling room from which the work crews began to dismantle and remove items and material that were then packaged in polypropylene bulk bags — commonly called supersacks — to meet U.S. Department of Transportation protocols for shipping contaminated material. The supersacks were lifted by cranes out of the Sturgis and loaded into special containers to be trucked to the designated waste disposal site operated by Waste Control Specialists in Andrews, Texas.
Various components — including a steam generator, coolant pumps, ductwork, valves, and piping — were removed first to provide access to the larger pieces of the reactor. Certain components were cut apart with diamond saws or other robust cutting tools. For example, there was a lead canopy atop the reactor containment vessel that had served as a safety barrier to protect personnel when the reactor was in use. The canopy was constructed in multiple layers and included an inner and outer layer of lead, plus interior materials consisting of asbestos sheeting and insulation, Barber says. “The team had to engineer a process to secure the multiple layers so that when we cut through the material and lifted the sections, the canopy would stay intact,” she explains. The canopy sections were lifted one-by-one out of the Sturgis by crane over a period of several months. The individual sections were scanned for radiological contamination and then double-bagged for storage on the deck of the Sturgis until it could be determined how much of the material might safely be recycled.
The primary shield tank was a ringlike system more than 9 ft tall that surrounded much of the reactor pressure vessel, its outer wall featuring an 8.5 in. thick annulus filled with lead shot. The tank was cut apart in three separate phases of work to minimize potential exposure to the crews, Barber says. The inner wall alone was cut into six sections and packaged in three separate bags.
When the initial work to cut apart the shield tank resulted in higher than anticipated radiological exposure for the cutting team, cameras and video monitors were set up inside the radiological zone to enable the cutting team to observe the cutting operations and minimize the employees’ exposure, Barber says. The system worked well enough that similar approaches will be used during the dismantling of the Fort Belvoir and Fort Greely reactors, she adds.
Other components from the Sturgis reactor remained mostly intact because of the potential for increased radiological exposure if they had been cut apart. These intact items could be quite large — for example, the spent fuel storage tank measured 27 ft tall and 8 ft in diameter and weighed more than 27 tons. The reactor pressure vessel — which once held the nuclear fuel and thus accounted for most of the radiological contamination remaining in the Sturgis — measured 13 ft tall and weighed roughly 81 tons. While still within the Sturgis, the reactor pressure vessel was secured inside a custom-fabricated shielded shipping container that measured more than 16 ft long and 10 ft wide. The shielded container was designed by EnergySolutions Inc., later acquired by Atkins, which is now part of SNC-Lavalin. After being carefully lifted out of the Sturgis, the shielded container was moved to the disposal site on a specially designed transporter constructed and operated by Perkins Specialized Transportation Contracting.
When the time comes, the reactor pressure vessels from the Fort Belvoir and Fort Greely reactors will also be secured within customized shielded containers — the design of each container dependent on the specific levels of radioactivity present in the components, Barber notes.
Once all the Sturgis reactor components had been removed, the remaining bulk of the barge was towed to International Shipbreaking Limited in Brownsville, Texas, where the former cargo ship/nuclear power plant vessel was cut apart for recycling and reuse. Over the years of the Sturgis reactor decommissioning project, more than 2.5 million lb of liquid and solid waste were safely removed and shipped to the disposal site, including more than 1.5 million lb of radioactive waste. More than 300 tons of lead and 5,000 tons of steel were safely recycled.
Through its work on the Sturgis, the Corps gained valuable insights into how to safely dismantle a nuclear reactor. For example, although the original estimated cost of the Sturgis project was $34.6 million, “the complexity of the project and a variety of unknowns encountered” during the work nearly doubled the final cost, which exceeded $65 million, Barber says. The engineering costs, in particular, were triple what the contractor had originally anticipated, she says, because of several factors, including:
- Not checking the engineering assumptions until implementation, which caused significant rework and project delays.
- Not ensuring that all engineering drawings were accurate and/or that the assumptions made about planning an activity were validated before starting the work.
- Learning that all engineering should not and cannot be done upfront for such complex efforts, as each phase may impact the next phase and could change the engineering approach.
- Not having an engineer on-site at all times to provide adequate support as new challenges arose.
The project also experienced problems because the team initially tried to perform the environmental abatement — of asbestos, lead, and other contaminants — on a piecemeal basis “as we worked our way to the reactor components,” Barber notes. But that approach raised too many safety and health issues. So for Fort Belvoir and Fort Greely, the contractors will be asked to fully remediate such potential hazards upfront.
The other two projects should also benefit from lessons involving relations with stakeholders, government officials, and the community. For example, the team intends to be even more proactive in engaging local government officials earlier in the planning process to ensure they understand the work planned for their communities. “Oftentimes, a great deal of coordination for projects may be done at the state level, with state-level coordinating agencies,” Barber says. “But with the Sturgis, it was the more local coordination with the elected officials and other stakeholders that helped build awareness and understanding of the project.”
Another key lesson was that such large, complex projects require close collaboration between the contractor and the government. “Daily interaction and cooperative team approaches to all work is a necessity,” Barber notes, adding that communication among members of the team “is paramount to success.”
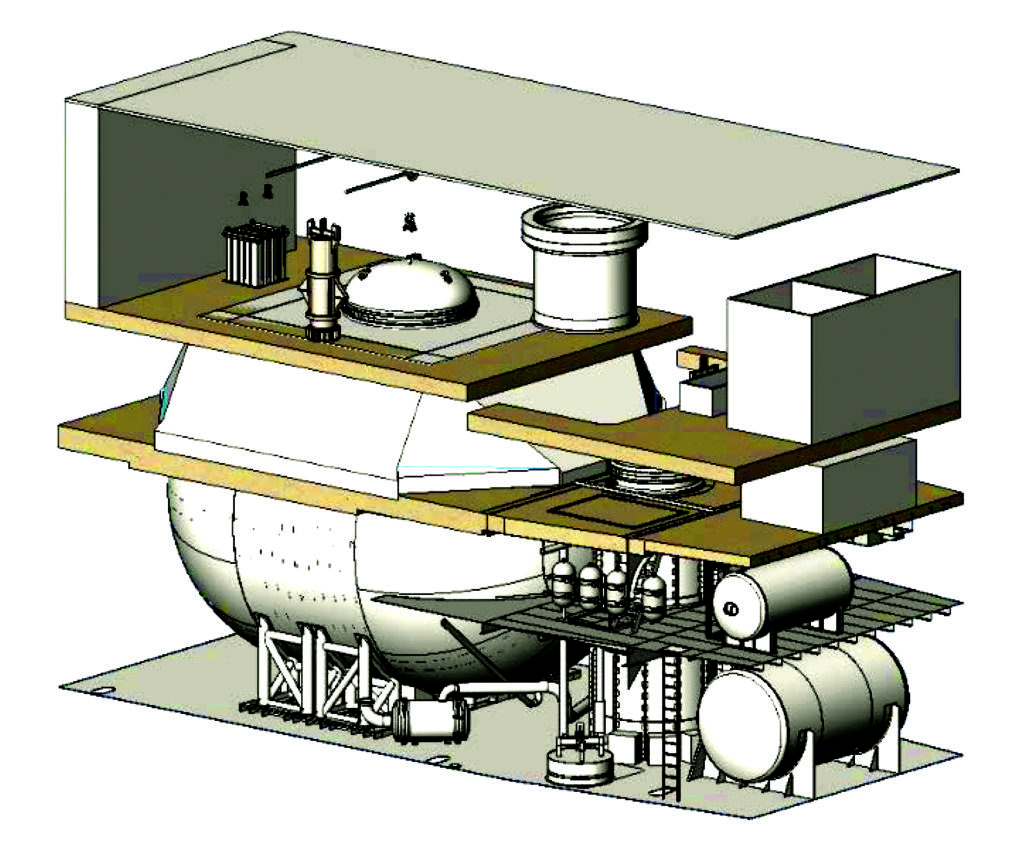
A lack of proactive communication with the local stakeholders on the Sturgis project turned out to be a key challenge, Barber says, especially because the mobility of the barge meant that there were multiple locations at which the work could take place. “When we actually awarded the contract and selected our final spot, we did have some communication challenges and some public relations issues that we learned some hard lessons from,” she adds. “So we are being very transparent and outgoing with respect to our communications for the other two projects. We’ve taken (the lessons from Sturgis) to heart.”
As for implementation, “we did have a lot of engineering challenges” during the Sturgis work, involving “how we approached the work, how we’d take things apart, what the best approach was to removing the components,” Barber says. “So we’ve taken those lessons learned and implemented some of that work for the next two reactors.”
Because the laser scanning and 3D modeling of the Sturgis work sites proved invaluable, a similar approach has already been completed for the Fort Belvoir project by the decommissioning planning team, a joint venture between AECOM and Tidewater, a Virginia-based firm that specializes in radiological cleanup, Barber says. The joint venture is helping develop a decommissioning plan, apply for the necessary permits, and perform other services. The actual dismantling and disposal work at Fort Belvoir will be conducted by a joint venture between AECOM and APTIM.
Because the Fort Greely reactor is partially encased in concrete — for protection against radiation because the site is a multifunctional facility, combining the power plant with a heating plant and other utilities — the laser scanning option is not available there. As a result, the work crews will need to use “other techniques for dose assessment and work planning,” Barber notes. The decommissioning team for Fort Greely has not yet been selected.
Fort Belvoir’s location along Gunston Cove meant that an intake and discharge system was used to pull in and discharge coolant water and to discharge effluent from the reactor operations. That system will be properly remediated and removed, Barber says, after which “we’ll survey the water and sediments to make sure there’s no contamination.” At Fort Greely, a similar system that relied on the nearby Jarvis Creek was removed in 1999 when the base was expected to be closed. The base remained opened, however, and so the early removal of the discharge line for the long-deactivated reactor “is one less thing for us to do when we actually decommission the site,” Barber notes.
At Fort Belvoir, the entire standalone structure housing the reactor will eventually be demolished, any contaminated soils will be removed, and new soil will be brought in as well as new vegetation, Barber notes. Because the Fort Greely site will continue to function as the base’s overall heating plant, only the reactor portion of that facility will be demolished. The site will be cleaned up, and the Corps will then restore the site and any necessary buildings required to support the base’s overall utilities.
In the future, Barber adds, the Army is considering the installation of new nuclear reactors at other remote locations. If that program moves ahead, one reason will be that the work on the Sturgis and at Fort Belvoir and Fort Greely proved the feasibility of removing such power systems at the end of their useful lives. “We successfully demonstrated that the reactors can be fully decommissioned,” Barber says. “We have the costs associated with that, and they have a full life-cycle picture of what it would take.” CE
PROJECT CREDITS Owner Army Deactivated Nuclear Power Plant Program Project management U.S. Army Corps of Engineers, Baltimore District Sturgis decommissioning planning IEM, now part of Plexus-NSD, Columbia, Maryland Sturgis decommissioning contractor CB&I Capital Services, now part of APTIM Federal Services LLC, Alexandria, Virginia Sturgis reactor pressure vessel shielded container EnergySolutions Inc., now part of SNC-Lavalin, Montreal Sturgis reactor pressure vessel shielded container transporter Perkins Specialized Transportation Contracting, Northfield, Minnesota Sturgis reactor dismantling site Malin International shipyard, Galveston, Texas Sturgis waste disposal Waste Control Specialists, Andrews, Texas Sturgis shipbreaking site International Shipbreaking Limited, Brownsville, Texas Fort Belvoir decommissioning planning AECOM-Tidewater Joint Venture, Alexandria, Virginia Fort Belvoir decommissioning contractor APTIM AECOM Decommissioning LLC, Alexandria, Virginia
This article first appeared in the December 2020 issue of Civil Engineering as “Nuclear Deconstruction.”