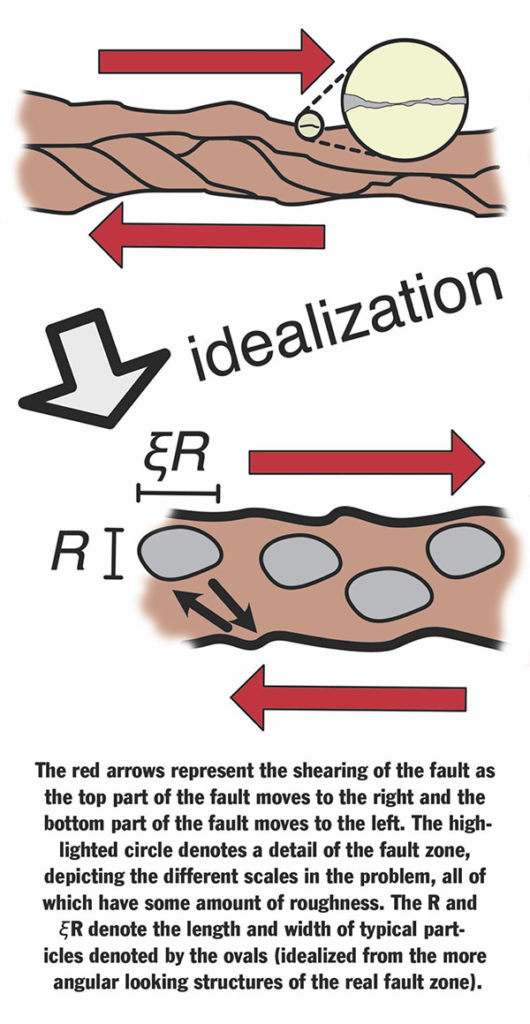
A NEW MATHEMATICAL model uses a landslide model and a pinball machine analogy to help predict the damage caused by ground motions during seismic events. The new model was developed by Victor C. Tsai, Ph.D., an associate professor of geophysics in the Department of Earth, Environmental, and Planetary Sciences at Brown University, and Greg Hirth, Ph.D., a professor of geophysics in the same department at Brown.
The new model is somewhat controversial, Tsai says, in part because it challenges the standard model of ground motions in earthquakes that has been used since the 1970s. That model states that when a seismic fault slips, it generates frictional failure that causes all the ground motion. Instead, according to a plain language summary of their paper, Tsai and Hirth argue that collisions of rocks within the fault zone “as they attempt to slide past each other during an earthquake may also create jerky ground motion.” Despite the importance of these fast, irregular motions, “most frictional models for earthquakes generally underpredict how strong they are,” Tsai and Hirth wrote.
Moreover, certain observed aspects of earthquakes — especially those involving high-frequency ground motions — are difficult to explain through the standard model, Tsai notes. High-frequency motions tend to cause most of their damage to smaller structures — those of five stories or fewer, for example — whereas low-frequency motions are more damaging to skyscrapers and other tall structures. Because the new model primarily predicts high-frequency ground motions, Tsai believes it should be used in conjunction with the standard model, not as a replacement for that standard approach.
The new model was developed on the basis of earlier research Tsai had conducted on debris flows and landslides. He was especially interested in the ground motions generated during wet landslides in which considerable amounts of debris are washed down a mountain after a storm. Building on that earlier work, Tsai and Hirth, who was not involved in the landslide research, studied the similarities and differences between debris flows and earthquakes to determine if a new model could predict similar types of ground motions. “The basic idea of our new model is that a fault zone — rather than being a simplistic planar structure — is more complicated, with all these geometrical heterogeneities” that include rocks ranging in size from less than 3 m across to more than 40 m, depending on the size of the fault zone and the earthquake of interest, Tsai says. The researchers wanted to know what happens to the rocks within the fault zone when the fault starts to move in an earthquake and the different “bits and pieces start to bang up against each other … what sort of ground motion would you predict from all the collisions between those different pieces?” Tsai asks.
Such collisions inspired his pinball analogy, Tsai notes, though the word “pinball” does not appear in any of the scientific papers. The basic concepts, however, have been around for decades in landslide studies, which is why he describes the application to earthquakes as a new contribution that “builds on literature that is 20 or 30 years old.”
Essentially, the new model should provide researchers with a different way to calculate how much ground motion to expect during a particular earthquake fault scenario. To do so, however, “you need to know something about the geometric structure of the fault zone, meaning you want to know how sharp or how rough or how smooth are the particles within the fault zone,” Tsai explains. “Once you know that, then you have a different way of predicting what the ground motions would be from that versus from the standard model of frictional slip.”
Such information on the fault zone can be derived from observations in the field. “You can go to exhumed faults which are faults at the surface currently and look at them and see how big the particles are and whether they are angular or very smooth,” Tsai says. But the challenge is that most faults around the world are not at the surface. “So then you need to use indirect observation,” he adds. “For example, sometimes people try to take seismic observations of the waves that occur during the earthquakes themselves, and you can use some of these same observations to try to infer certain aspects of the fault zones, including potentially how rough they are.”
Then, “if you knew that a fault zone had angular particles, that would predict a certain amount of ground motion from our model,” Tsai says. “And if you also knew that there was this low stress-drop — meaning a low amount of stress built up on that fault — then the standard model would predict a very different result.”
Earthquake engineers need to know whether “we have a good understanding of what creates the ground motions that cause damage in buildings,” Tsai says. To that end, “there are certain places around the world where we know now, based on previous observations, that the earthquakes there tend to have higher ground motions than at other places,” he adds. “So our work potentially helps us better understand that aspect — where and for which faults you would expect there to be larger ground motions versus smaller ground motions.” As a result, earthquake engineers and others “might want to concentrate their energies” on enhancing the building codes in those higher ground-motion locations.
To date, many earthquake ground-motion models have been purely empirical, trying to make the existing data fit various curves — for example, using past earthquake data to estimate the extent to which shorter distances are correlated with stronger shaking, Tsai says. “But there is no physics in many of these empirical fits,” he explains. “Therefore, they may miss a lot of interesting behavior, such as earthquakes at certain fault zones having generally stronger shaking compared with those at other fault zones.” The pinball model is based on trying to better understand the physics that create these ground motions. “We think that’s actually quite crucial to being able to better predict damage from future earthquakes,” Tsai explains.
The new model must still be verified, Tsai adds. At press time, he was about to begin additional research in three areas in which the new model makes distinctly different predictions from the standard frictional model — areas that are potentially observable and thus “might be good enough to really test the two types of models,”
he says. —Robert L. Reid
This article first appeared in the October 2020 issue of Civil Engineering.