Engineers are known for the kinds of innovative, “blue-sky” thinking that have resulted in mobile phones and driver-assistance technology, but such creative approaches are not always feasible in the field of structural engineering. It is for the most part a profession that relies heavily on formulas and building codes. But even within the confines of structural engineering, there is room for innovation and outside-the-box thinking. That is what happened with the design of the Başakşehir Pine and Sakura City Hospital in Istanbul. The Arup-led design team established project-specific seismic performance objectives aligned with the owner’s risk tolerance, explored structural system alternatives that met these performance objectives, and delivered an optimized design on time.
In 2009, Turkey’s Ministry of Health launched the New City Hospitals program and promoted it by using a public-private partnership model. The program’s objective was to build large public hospitals in multiple cities to provide increased access to modern health care facilities and drive medical tourism in Turkey. Depending on the projected demands in bed capacity for each city, these new hospitals would either replace one or more of the existing hospitals in the cities or add to them.
As part of this investment project, 11 city hospitals have opened since 2017. Common features of these new medical facilities are a significantly higher number of beds and intensive care units, smart building automated systems, and technologically advanced medical equipment. In addition, the new city hospitals that are located in seismic regions are base isolated — a design feature that enhances their seismic resiliency by providing continuous functionality after a major earthquake.
Başakşehir Pine and Sakura City Hospital was the most recent hospital built as part of this project. Located in the highly seismic city of Istanbul, which has a population of more than 15 million, the hospital has a total area of 10 million sq ft and features more than 2,000 seismic isolators. It is the largest base-isolated structure in the world, and there are no seismic joints within the building.
Located only 20 mi from the Istanbul Airport, the hospital aims to make the city the health care hub of the surrounding region. To provide ease of access to the hospital, the Ministry of Transport and Infrastructure invested in an approximately 4 mi long new metro line to connect the hospital to the nearest metro station.
The hospital was built by Rönesans Holding and Sojitz Corp., a Japanese investment and trade corporation. The architectural concept was prepared by Perkins&Will, and Yazgan Design Architecture and Hayalgücü Design served as the project architects. Arup developed the seismic design of the base-isolated superstructure, podium, and foundations, and local firms EFK Mühendislik, Mista Mühendislik İnşaat San. ve Tic. Ltd. Şti, and APCB were responsible for the gravity system design. Kilci Mühendislik, located in Ankara, Turkey, was the geotechnical engineer, and OBS, of Istanbul, was the seismic consultant.
The base-isolated main hospital facility is 350 ft tall and consists of three specialty towers that are 14, 16, and 17 stories; three helipads; and six clinic buildings — all of which share a five-level common podium with no seismic joints. The podium beneath the towers and the clinics is supported on 2,068 seismic isolators. Parking, with more than 8,000 spaces, is allocated within the underground portion of the podium at the lower three levels. By locating all the parking underground, 2 million sq ft was made available for landscaping that features cherry trees irrigated by a rainwater harvesting system.
Concept design began in the second quarter of 2016, and the structural design was complete within a year. Construction at the site started in 2017 and finished in 32 months. The hospital had its partial opening of 1,700 beds in April 2020 in support of the COVID-19 response in Istanbul. The grand opening took place a month later, and the hospital is now in full service to about 32,700 patients per day, with 90 operating rooms and 458 intensive care units. The new hospital has added 2,682 beds to the city’s health care system.
The hospital was constructed on a greenfield site in the developing district of Başakşehir, with no disruption to any of the surrounding residential buildings or infrastructure.
Site location and seismic performance
The geotechnical engineer determined three soil profiles along the footprint of the podium at the foundation levels, with shear wave velocities ranging from 650 ft/second to 2,000 ft/second. Some locations required fill material. The use of shallow foundations for a base-isolated building in such soil conditions would not have been feasible, so the geotechnical engineer recommended pile foundations. The foundation system consists of 7,500 piles that are 40 in. in diameter and vary in length between 16 and 100 ft, with 2,000 pile caps.
The project site is located 15 mi north of the closest fault trace, which is part of the North Anatolian Fault system in the Marmara region. The site-specific seismic hazard parameters are SDS = 1.15 g and SD1 = 0.82 g. (SDS is the design response acceleration parameter at short periods; SD1 is the design response acceleration parameter at a period of 1 second; and g is acceleration due to gravity.) The Turkish code allows base-isolated structures to be designed with limited ductility; therefore, the superstructure of a base-isolated hospital building can be designed using a response spectrum analysis with a response modification factor, R, of 1.2. This satisfies the immediate occupancy building performance objectives under the design basis earthquake (BSE-1N).
Per the same code, the isolation plane is required to satisfy immediate occupancy performance criteria under the maximum considered earthquake (BSE-2N).
To achieve enhanced seismic performance objectives, the hospital was designed to satisfy the ASCE 41 immediate occupancy performance objective under a very rare earthquake (BSE-2N) with a drift limit of 1 percent and an operational performance objective under the design basis earthquake (BSE-1N) with drifts limited to 0.5 percent. Floor accelerations were limited to 0.2 g to minimize damage to nonstructural components and achieve a resilient building design that would continue to operate fully after a major earthquake.
Concrete optimization
The gravity system comprises reinforced-concrete slabs and beams supported by cast-in-place concrete columns. Typical grids are 8.4 by 8.4 m, and the floor-to-floor heights vary from 4 to 6.5 m, with the typical floor height being 4.3 m. The lateral load-resisting system consists of special reinforced-concrete walls that resist 100 percent of the lateral loads in both orthogonal directions of the building.
Given the size of the project, a core wall-only lateral system offered the best potential for varying story heights and optimization of concrete and reinforcement quantities. Therefore, Arup conducted an extensive wall optimization study to minimize construction costs and improve floor plan efficiency. The study aimed to optimize the concrete quantity while providing sufficient lateral stiffness to the base-isolated building. The design team used a fixed-base model of the structure in the study, with the assumption that the structure needed to have one-third to one-half of the period of the base-isolated building. This would ensure dynamic decoupling between the superstructure and the isolation system.
The study examined five wall thicknesses —16 to 32 in., in 4 in. increments. The walls were grouped into nine categories based on their plan geometry and height. Given these two input components, the various wall thicknesses, and nine wall groups, one could arrive at roughly 2 million wall thickness layouts. This total included layouts that were structurally unacceptable or impractical, such as thinner wall thicknesses at the lower floors and thicker ones at the upper floors.
Therefore, the design team employed a logic filter to eliminate the unacceptable or impractical combinations, which reduced the number of possible configurations to 180. This was still a large number of models to be generated and analyzed, so the design team established a workflow such that all 180 models were generated and analyzed automatically. The resulting data were stored in a database with images of modal properties of each model.
Arup created an interactive web platform that enabled Rönesans Holding to have access to the modal analysis results of all the models and the associated concrete volumes. This allowed for client engagement in the decision-making process and facilitated the selection of the optimum concrete wall thicknesses. The most efficient system resulted in 70,000 cu m of special concrete walls.
Nonlinear time history analyses were performed using LS-DYNA software. Over the past decade, Arup developed a workflow for LS-DYNA analyses that features automated model generation, multiple concurrent analyses, and postprocessing via cloud computing. With a cloud-based workflow, it is possible to run large suites of analyses in parallel, saving a significant amount of time. LS-DYNA has a large library of material models that accurately capture the nonlinear behavior of reinforced-concrete elements.
The hospital’s concrete walls were modeled using composite layered shell elements available in LS-DYNA, using Mat Concrete EC2, which is a concrete material model developed by Arup. The concrete walls, coupling beams, and isolators were modeled inelastically. The hospital’s gravity system as well as the isolation plane elements — concrete beams tying the isolators together and the concrete pedestals — were modeled as elastic elements.
Evaluation of the isolation system
Selection of the isolation system was of utmost importance because the procurement of the isolators was a critical path item for the design and construction processes and a primary driver that influenced the overall construction cost. Arup supplied the client with the building performance objectives under a range of isolator types to facilitate the selection of the optimum type and layout for the project. Arup conducted an isolation scheme optimization study in which three types — triple-friction pendulum bearings, lead-rubber bearings, and high-damping rubber bearings — were studied, including their respective impacts from the superstructure to the foundations.
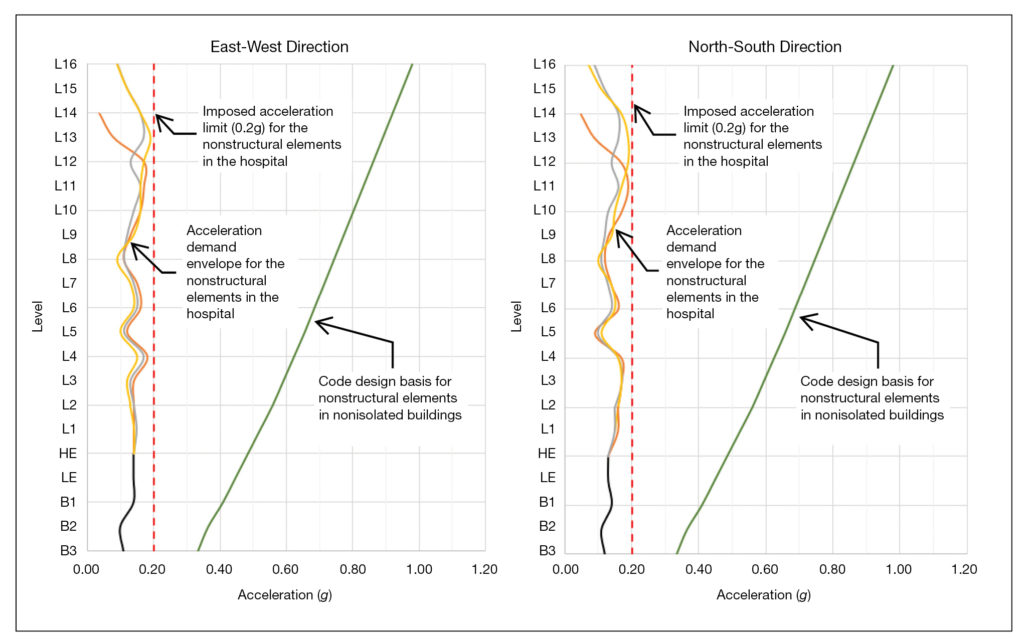
These isolators were evaluated with various layouts, resulting in six isolator schemes. Six nonlinear models of the entire building were generated and evaluated under seven pairs of horizontal and seven vertical time histories — both for upper-bound and lower-bound isolator properties — which resulted in 168 time-history analyses. Arup presented the results of the superstructure and isolation-level evaluation to Rönesans, which opted to use the TFP bearings. The final design ensured the hospital could displace by 27 in. during an earthquake at the isolation level, minimizing damage to the components of the superstructure.
For the Başakşehir Pine and Sakura City Hospital, using local building code provisions would have been sufficient to obtain a construction permit. However, Arup informed the client of the importance of selecting project-specific seismic performance objectives with a clear focus on rigorous optimized structural design — driven by constructability and procurement objectives — which can make the delivery of a cost-efficient project within the same schedule achievable.
Structural engineers have a unique opportunity to contribute to the improvement of resiliency in their cities by establishing dialogues with the various stakeholders to set project-specific seismic performance targets. These metrics may include safety, level of repairs and/or downtime, and business continuity after an earthquake. To achieve these targets while optimizing the design, it is essential to conduct rigorous and broad-spectrum concept explorations in conjunction with comprehensive analyses.
For now, early client communications on seismic performance objectives and rapid exploration of alternative solutions could be the only answer to how we can achieve improved resiliency in our communities — one building at a time.
PROJECT CREDITS Client/Owner/Construction Rönesans Holding, Ankara, Turkey Investment Rönesans Holding, Ankara, and Sojitz Corp., Tokyo Architectural concept design Perkins&Will, Washington, D.C., office Project architects Yazgan Design Architecture, Ankara, and Hayalgücü Design, Ankara Seismic design Arup, Los Angeles office Gravity system design EFK Mühendislik, Ankara, Mista Mühendislik İnşaat San. ve Tic. Ltd. Şti, Istanbul, and APCB, Ankara Geotechnical engineer Kilci Mühendislik, Ankara Seismic consultant OBS, Istanbul
SIDEBAR
Structural engineering in the cloud
By Atila Zekioglu, P.E., S.E., M.ASCE, and Kermin Chok, P.E., S.E.
Structural engineering analysis tasks have always been computationally demanding. For many decades, structural engineers depended on their abilities to simplify complex 3D structural systems into a series of 2D representations or even 1D approximations. With the development of more robust computing, the analysis and validation of the engineer’s intuition and experience-based ideas were soon based on algorithms and limited only by the available computational processing speed. But even then, costs were significant and the investment in time was best measured in weeks.
Even with the sweeping improvements we have seen in available computing power, speed is still an important factor in the design schedules associated with projects. The number of alternate structural system options has always had to be balanced against the complexity and fidelity of the structural analysis models themselves.
Nonlinear analysis was the driver for Arup’s journey into cloud computing, but the benefits for all structural workflows are clear.
Now, however, with the confluence of parametric design and cloud computing, that balance point can and should be reconsidered. With access to previously unimaginable computing resources and managed cloud services for storage and databases, the profession is at a unique juncture at which it can reimagine how structural engineers explore creative concepts and compare the performance of various system options. Results can be returned within hours, meaning projects can be delivered far more swiftly for clients and communities.
The nonlinear time-history analysis used in the seismic realm is perfectly suited to benefit from this democratization of computing resources. One- and two-dimensional solutions may be things of the past, but seismic problems are still often simplified to static or dynamic analysis methods using elastic theory to manage and reduce computational intensity. This can be inappropriate for building systems with highly dynamic nonlinear responses and in structures displaying both geometric and material nonlinearities. (Geometric nonlinearity occurs when a building moves in many directions during an earthquake and does not retain its pre-earthquake geometric position, as most analyses assume. Material nonlinearities occur when structural steel and reinforced-concrete elements go beyond their elastic capacities during an earthquake, resulting in permanent deformations.)
Arup has a long history with the advanced, general-purpose, multiphysics simulation software package LS-DYNA and with in-house coding of custom material models for the package. LS-DYNA analytical models have a high level of fidelity, require evaluation of the structure over a large suite of earthquake excitations, and generate a significant amount of raw binary data.
Arup has used this system along with commercial cloud computing services to produce innovative designs for two recent projects: the Başakşehir Pine and Sakura City Hospital in Istanbul and the (W)rapper, a high-rise office building in Culver City, California.
With cloud computing, the nonlinear response history analyses (NLRHA) for each of these finite element models take about 8 hours to solve using 28 computer cores. Furthermore, because performance-based seismic design requires the evaluation of the structure under numerous ground motions ranging from 64 to 110 earthquake simulations, the ability to solve all these models in parallel leveraging the elasticity of the high-performance computing environment is invaluable. In total, each analysis and design iteration would thus have required on the order of 14,500-plus core hours of simulation. In the past with traditional on-premise computing limitations, these suites of analysis would have taken weeks to execute.
This project illustrated the need for a clean data transfer from various software and modeling domains. Without the advances and availability of cloud computing, these workflows would not have been feasible within the project timeline.
However, using Arup’s high-performance computing cloud provider, the company was able to turn around these simulations overnight. All analyses were solved in parallel, as opposed to the weeks that this would traditionally take if they were attempted sequentially using in-house hardware. In-house training and seismic analysis-specific tools and templating have smoothed the transition from running the simulations in-house to running them in the cloud.
Coupled with the parallel computational system was custom cloud architecture for the storage and postprocessing of the terabytes of binary and numerical data the processes generated. Visualizations and reports were generated automatically, making the designs easier to share among engineering team members. Dashboards built on top of this cloud data infrastructure facilitated further dissemination of the results to the wider project team and enabled client engagement in the design process.
For the (W)rapper building, an architecturally integrated structural system of exoskeleton steel bands coupled with high seismic demands resulted in a lateral-force-resisting seismic system of steel bands and steel-plate shear walls. Base isolation was used to ensure that the building’s seismic performance was adequate at the maximum considered earthquake level. To incorporate these elements, the tower was analyzed using the NLRHA method.
The geometrically complex model was generated parametrically using Rhino-Grasshopper. A suite of digital components developed in-house were used to facilitate the precise control of the geometry and the assignment of the nonlinear materials across the geometrically complex analysis model. Such automation freed the design team from manual bookkeeping and allowed more time to study this complex structural system and its components.
The design team further leveraged the tool set to create higher-fidelity, finite-element shell models of portions of the structure, which were then subjected to a time-history excitation to study local plate-buckling modes and stress-strain histories.
This project illustrated the need for a clean data transfer from various software and modeling domains. Without the advances and availability of cloud computing, these workflows would not have been feasible within the project timeline. The project team leveraged commercial cloud resources to process the approximately 16 terabytes of binary NLRHA results while using big-data platforms to rapidly query, aggregate, and transform the time-domain results into actionable engineering information.
Nonlinear analysis was the driver for Arup’s journey into cloud computing, but the benefits for all structural workflows are clear. Arup is now putting cloud-native structural workflows back into the linear elastic realm to further aid in architectural optioneering and rapid scheming in real time. In the linear elastic optioneering realm, structural analysis models can run in minutes instead of hours, and result sets can range in gigabytes rather than terabytes. This reduction in analysis complexity allows for further exploration and expansion of the design space.
Arup is using these cloud-native workflows to enable fully parametric analysis and building information model generation, web-based “drag and drop” model postprocessing, and project-agnostic optioneering. The balance point between model fidelity and the number of options that can be explored has shifted. The result is a more complete exploration of the possible design space and better engineering solutions.
This article first appeared in the March/April 2021 issue of Civil Engineering as “Performance-Based Seismic Design Succeeds in Turkey.”
Very good news for countries that have suffered a lot from earthquakes. Congratulations, hocalarım. Technology saves lives.
Excellent, much informative & knowledge sharing the projects briefing along with lessons Learned & how it overcome is outstanding.